Publications
Selected Publications
Abstract
The study of variation in biomechanical properties is a significant diagnostic tool for examining malignancy in tissues. Elastography is a potential technique that is used for qualitative and quantitative assessment of abnormal tissues. Optical coherence elastography is a highly efficient and promising technique for inspecting soft biological tissues with microscale resolution. In the present work, we report the elastographic measurement of tissue-mimicking phantoms of varying stiffness using reflection mode holographic imaging. The sinusoidal surface excitation is produced by an electromechanical actuator on the sample over a set of frequencies and the phase map of the surface wave is reconstructed using a phase-shifting algorithm. The preliminary results are well correlated with previously reported literature. The current work has high potential applications for quantifying the biomechanical properties of in-vivo and ex-vivo tissues in clinical practice.
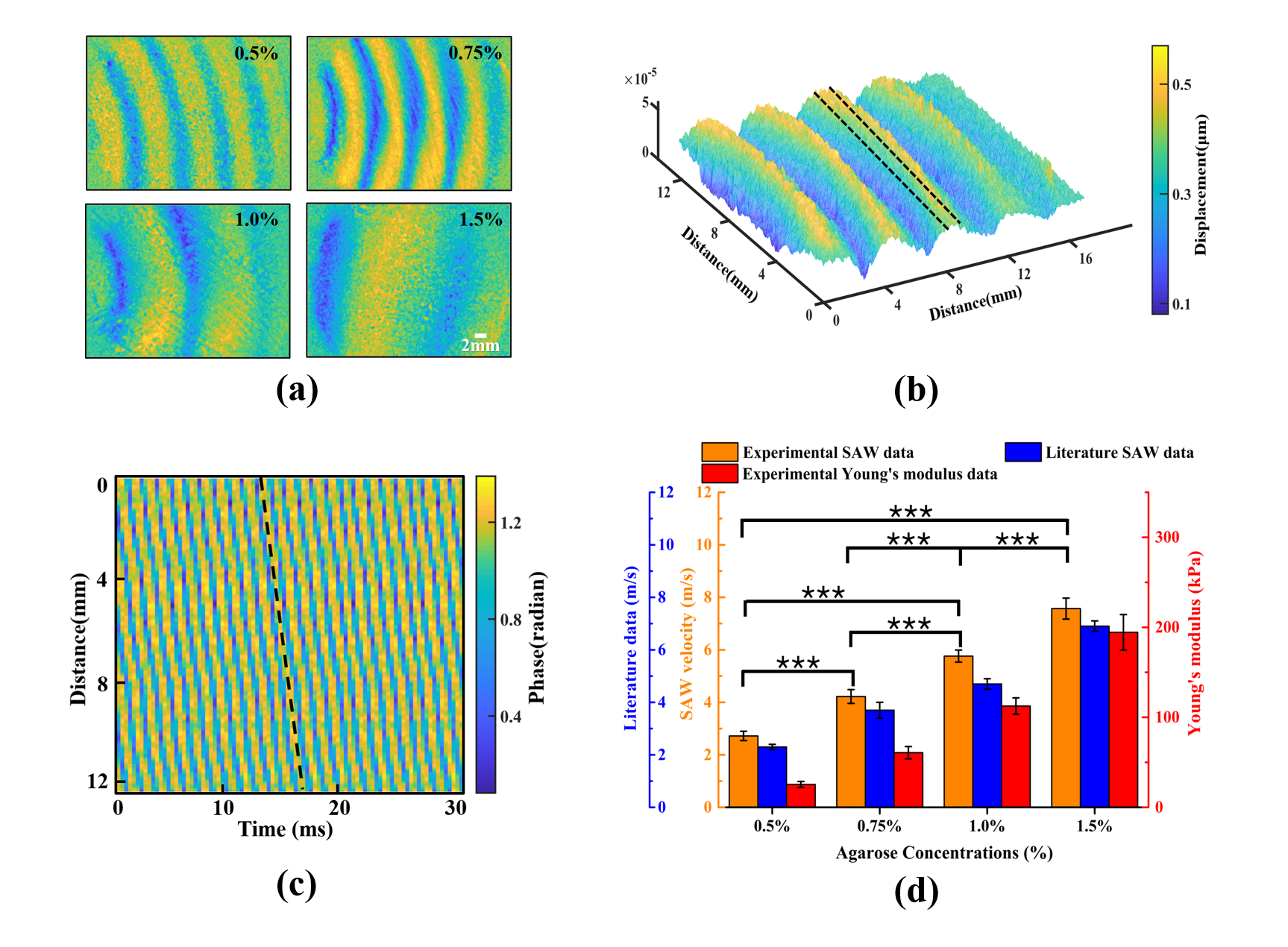
Abstract
Single-shot reconstruction of the inline hologram is highly desirable as a cost-effective and portable imaging modality in resource-constrained environments. However, the twin image artifacts, caused by the propagation of the conjugated wavefront with missing phase information, contaminate the reconstruction. Existing end-to-end deep learning-based methods require massive training data pairs with environmental and system stability, which is very difficult to achieve. Recently proposed deep image prior (DIP) integrates the physical model of hologram formation into deep neural networks without any prior training requirement. However, the process of fitting the model output to a single measured hologram results in the fitting of interference-related noise. To overcome this problem, we have implemented an untrained deep neural network powered with explicit regularization by denoising (RED), which removes twin images and noise in reconstruction. Our work demonstrates the use of alternating directions of multipliers method (ADMM) to combine DIP and RED into a robust single-shot phase recovery process. The use of ADMM, which is based on the variable splitting approach, made it possible to plug and play different denoisers without the need of explicit differentiation. Experimental results show that the sparsity-promoting denoisers give better results over DIP in terms of phase signal-to-noise ratio (SNR). Considering the computational complexities, we conclude that the total variation denoiser is more appropriate for hologram reconstruction.
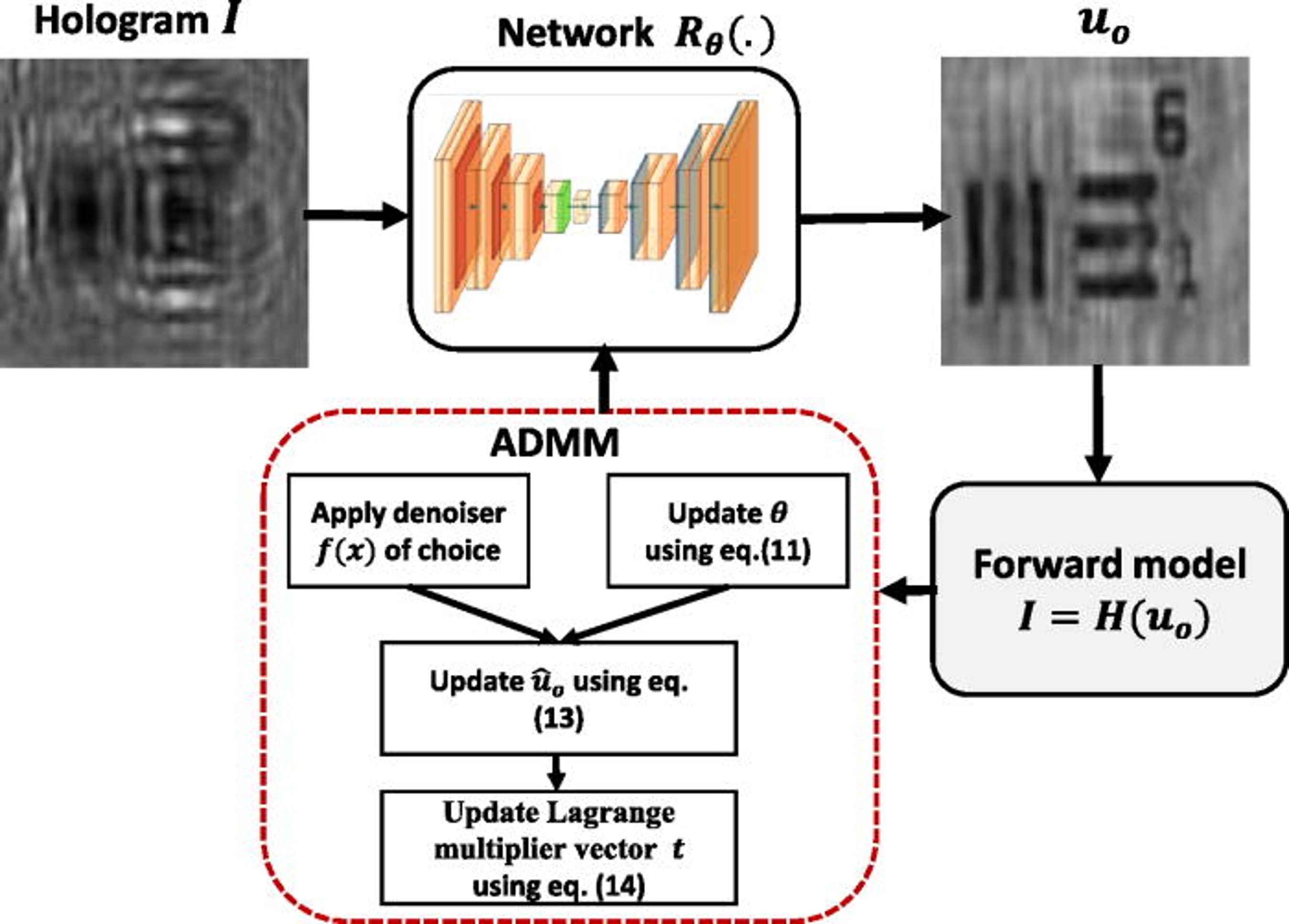
Abstract
Viscoelasticity is an important diagnostic parameter to investigate physiological dysfunctions in biological tissues. This Letter reports the quantification of viscoelastic parameters by Rayleigh wave tracing on the surface of tissue-mimicking phantoms using holographic imaging. The Rayleigh wave is induced by an electromechanical actuator on the surface of oil-in-gelatin phantoms and a biological tissue sample followed by holographic imaging and reconstruction of the wave. The frequency-dependent velocity dispersion is fitted to a Voigt model for the quantification of viscous and elastic moduli. The viscoelastic parameters calculated by the proposed method are validated by comparing the results from a conventional mechanical rheometer.
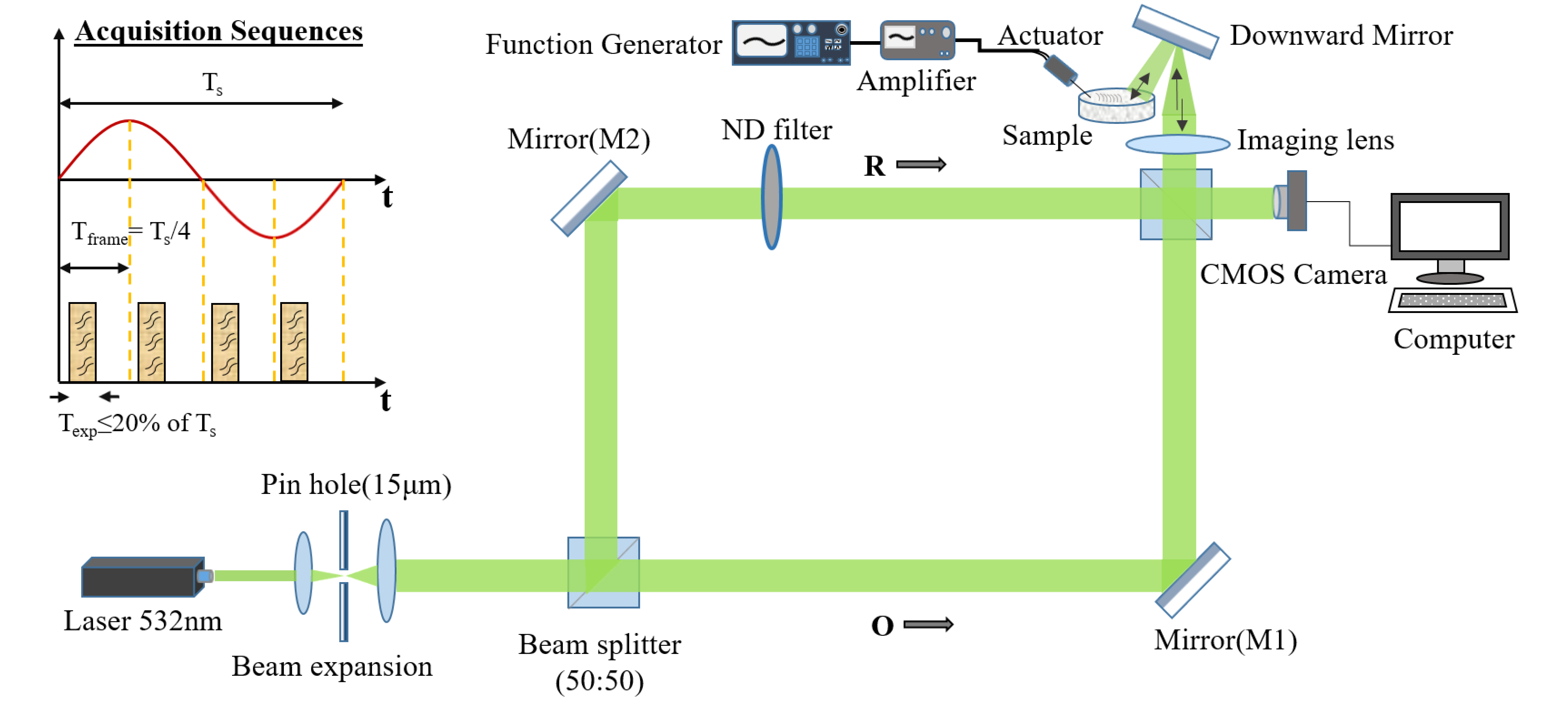
Abstract
The twin image-free phase reconstruction is still a challenge with single-shot inline holographic systems. Existing solutions mostly are based on the inverse problem approaches or alternating projections. However, there exists a trade-off between phase retrieval and twin image elimination. Recent studies have introduced a hybrid method involving both the approaches to mitigate this trade-off. Following these works, we propose a single-shot sparsity-assisted iterative phase retrieval approach that applies a sparsity constraint in the object domain and formulates phase retrieval as a minimization problem. We demonstrate lensless digital inline holographic microscopy for imaging transparent and weakly scattering biological samples over a large field-of-view of 29mm2. The proposed method achieves high fidelity phase reconstruction with faster convergence compared to the existing single-shot phase retrieval methods. We further demonstrate the phase quantification of label-free biological samples, such as cervical cells and RBCs, to highlight the potential of our technique in clinical applications.
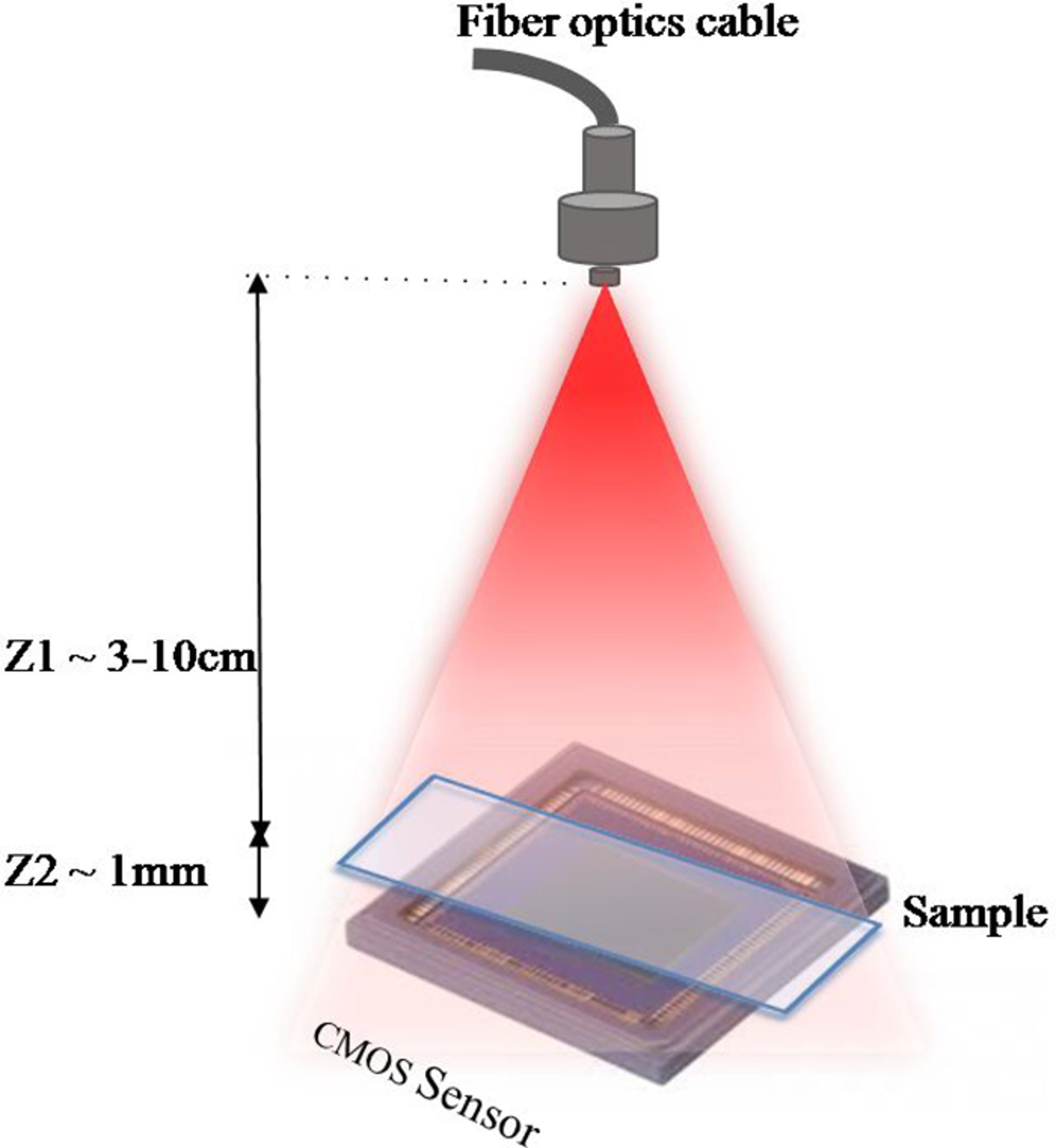
Abstract
Presence of a range of insects with different shape, size, and structural morphology of compound eye reveals the diverse pathways adopted by the nature striving to develop a flawless vision under given set of constraints. Exploration of various compound eyes allows researchers to understand better the various stages of visual system development in insects. By far, the most frequently used techniques for the investigation of structural morphology of a compound eye are scanning electron microscopy, transmission electron microscopy, microcomputed tomography, and histology. The nature of implementation of these techniques restricts in vivo studies, and also the requirements for a complicated and costly setup put limitations on its availability at small research facilities. Despite the widespread acceptance of the optical coherence tomography (OCT) as a standard tool for the various ophthalmological investigations, reports on compound eye study using OCT are uncommon. We demonstrate the application of a full-field OCT (FF-OCT) system for depth-resolved structural imaging and morphological analysis of the compound eye dioptric system. We report the application of a time-domain FF-OCT system for depth-resolved en face and three dimensional in vivo imaging of dragonfly’s prominent compound eye with resolutions of 2.8 and 7.2 μm in lateral and axial directions, respectively. The study shows the effectiveness of the FF-OCT approach for label-free and rapid structural morphology analysis of compound eyes. The results have been compared with the histology results reported in literature.
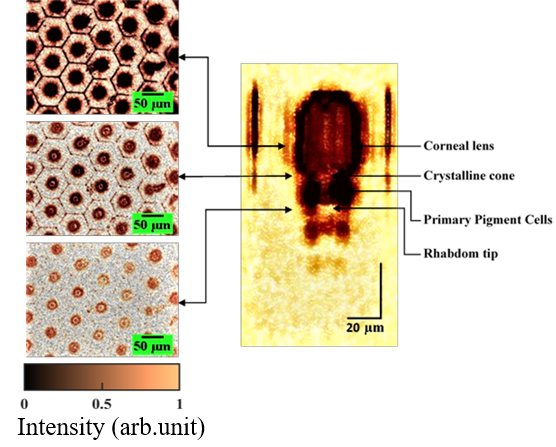
Abstract
The assessment of mechanical stiffness is an essential diagnostic tool for investigating the biomechanical properties of biological tissues. Surface wave elastography (SWE) is an emerging technique to quantify elastic properties of tissues in clinical diagnosis. High-speed optical imaging combined with SWE has enormous potential in quantifying the elastic properties of tissues at microscale resolutions. In this study, we implement surface wave elastography using high-speed optical interferometry to characterize the elastic properties of tissue-mimicking phantoms and ex-vivo native caprine liver tissue by imaging the surface wave induced by an electromechanical actuator. The sinusoidal mechanical excitations ranging from 120 Hz to 1.2 kHz on the surface of tissues are captured using a high-speed camera with a frame rate of 4 kHz at micrometer resolutions. The surface wavefront reconstruction is performed using a phase-shifting algorithm and linear regression is used to calculate the surface wave velocity. The mechanical stiffness estimated from the optical system is compared with the results of mechanical compression testing measurements. The results from this multimodal platform combining optical interferometry and vibrational spectroscopy using SWE are highly promising towards a non-invasive or minimally invasive imaging for in-vivo and ex-vivo mechanical characterization of tissues with future clinical applications.
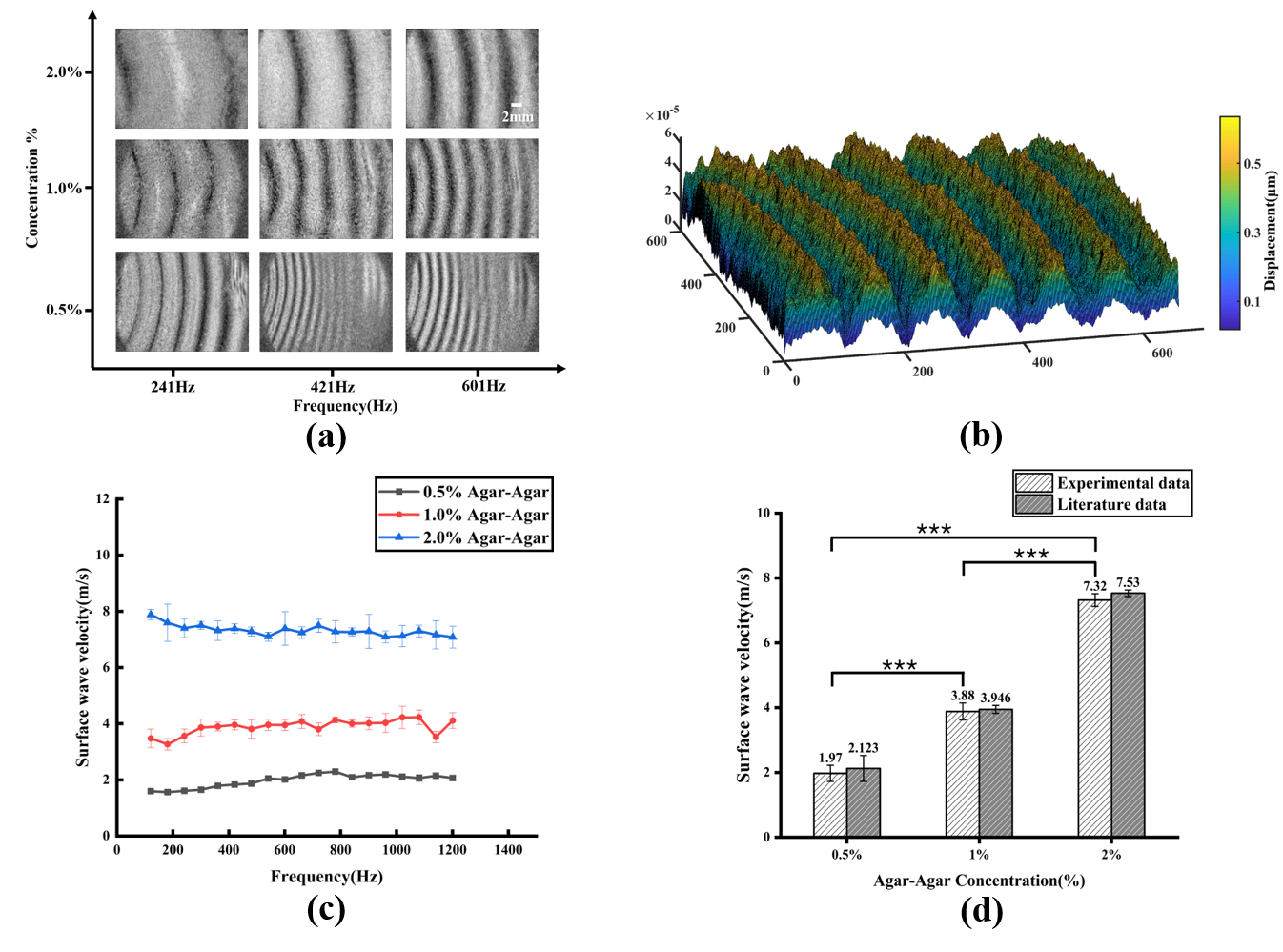
Abstract
Conventional cytology is a rapid chair-side method for diagnosis, but it relies on laborious fixing and staining protocols. As cytology specimens are transparent, it is very hard to visualize them under a bright-field microscope without staining. Quantitative phase imaging techniques have opened up an interesting and potential diagnostic method for volumetric three- dimensional (3-D) visualization of the transparent specimens without any need for sample prep- arations. We explore the use of digital holographic microscopy in clinical application of oral cytology for the 3-D visualization of buccal cells with high contrast without any additional sam- ple preparations. We also propose nuclear to cytoplasmic (N∕C) volumes as a much more accu- rate parameter for identification of multinucleate and actively dividing cells. We quantify the cellular volumes, and N∕C ratios for 203 buccal cells taken from five healthy volunteers to clinically validate the technique and compare them with the traditional N∕C area ratios as well as the histology standards. The mean N∕C area and volume ratios are found to be 0.0322 0.0149 and 0.0648 0.0286, respectively. Our approach highlights the dawn of a new method for a label-free/nondestructive volumetric oral cytology evaluation, with high potential for explo- ration of suspicious oral lesions, in subjects with chronic habits such as alcoholism and tobacco use.
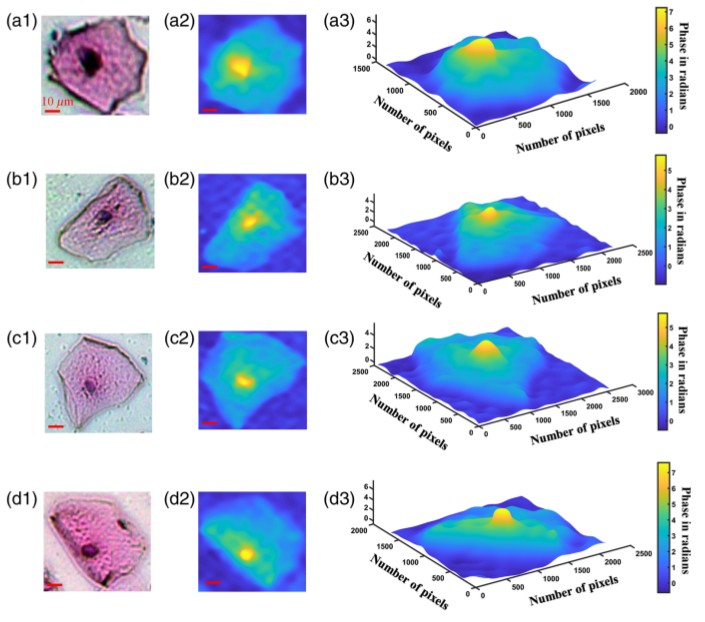
Figure: Label versus label-free evaluation: a comparison between (a1)–(d1) label/stained versus (a2)–(d2) label-free QPI images is shown for four buccal cells, with an additional 3-D volume view generated through phase retrieval shown in (a3)–(d3). The accuracy of our method can be well appreciated, comparable with to H&E-stained images.
Abstract
We present results from non-interferometric quantitative phase imaging (QPI) for thickness estimation and morphological analysis of human spermatozoa. QPI along with algorithms for image processing enable localization and quantification of subcellular structures of spermatozoa, including the acrosomal, nuclear, mid-piece, and tail regions. This approach based on the transport of intensity equation (TIE) using a simple brightfield microscope renders quantitative phase image with nanometric depth sensitivity, allowing the precise subcellular structural resolution of human spermatozoa. We demonstrate the potential of TIE in real-time phase imaging and the morphological analysis of spermatozoa. We first calibrate the TIE using microbeads and a standard phase target of known dimensions. Image processing for quantitative volume estimation of nuclei and acrosomal regions is carried out on the reconstructed quantitative phase images. We also apply a Hilbert transform-based image processing algorithm to the retrieved phase for visualization of vacuoles present on the sperm head.
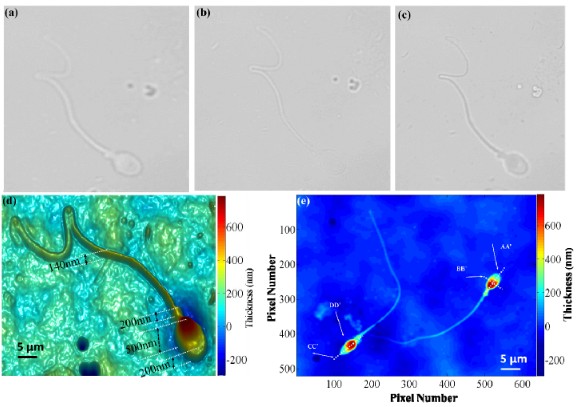
Abstract
This paper demonstrates an ultrasensitive microfluidic biochip nanoengineered with microporous manganese-reduced graphene oxide nanocomposite for detection of cardiac biomarker, namely human cardiac troponin I. In this device, the troponin sensitive microfluidic electrode consisted of a thin layer of manganese-reduced graphene oxide (Mn3O4-RGO) nanocomposite material. This nanocomposite thin layer was formed on surface of a patterned indium tin oxide substrate after modification with 3-aminopropyletriethoxysilane and was assembled with a polydimethylsiloxane-based microfluidic system. The nanoengineered microelectrode was functionalized with antibodies specific to cardiac troponin I. The uniformly distributed flower-shaped nanostructured manganese oxide (nMn3O4) onto RGO nanosheets offered large surface area for enhanced loading of antibody molecules and improved electrochemical reaction at the sensor surface. This microfluidic device showed an excellent sensitivity of log [87.58] kΩ/(ng mL–1)/cm2 for quantification of human cardiac troponin I (cTnI) molecules in a wide detection range of 0.008–20 ng/mL. This device was found to have high stability, high reproducibility, and minimal interference with other biomarkers cardiac troponin C and T, myoglobin, and B-type natriuretic peptide. These advantageous features of the Mn3O4-RGO nanocomposite, in conjunction with microfluidic integration, enabled a promising microfluidic biochip platform for point-of-care detection of cardiac troponin.
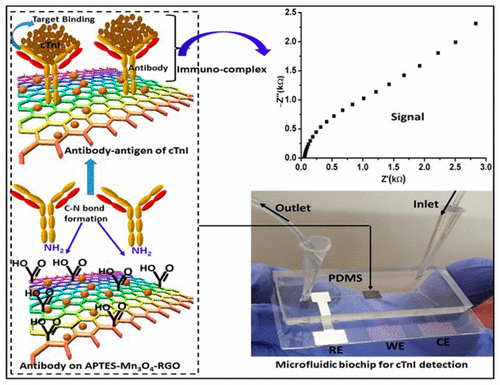
Details
(a) Formation of protein-corona on the surface of mNPs@rGO sheets. (b and c) G-Pd@rGO nanocomposite, inset of (c) shows a high-resolution image of G-Pd@rGO, (d) G-Pt@rGO nanocomposite, (e) magnified image of G-Pt@rGO nanocomposite. (f and g) G-Ag@rGO nanocomposite and (h and i) G-Au@rGO nanocomposite.
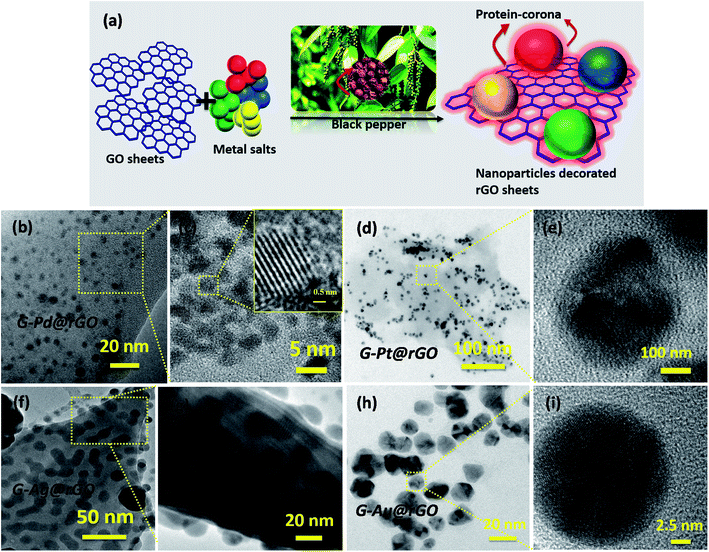
Abstract
We propose a versatile 3D phase-imaging microscope platform for real-time imaging of optomicrofluidic devices based on the principle of digital holographic microscopy (DHM). Lab-on-chip microfluidic devices fabricated on transparent polydimethylsiloxane (PDMS) and glass substrates have attained wide popularity in biological sensing applications. However, monitoring, visualization, and characterization of microfluidic devices, microfluidic flows, and the biochemical kinetics happening in these devices is difficult due to the lack of proper techniques for real-time imaging and analysis. The traditional bright-field microscopic techniques fail in imaging applications, as the microfluidic channels and the fluids carrying biological samples are transparent and not visible in bright light. Phase-based microscopy techniques that can image the phase of the microfluidic channel and changes in refractive indices due to the fluids and biological samples present in the channel are ideal for imaging the fluid flow dynamics in a microfluidic channel at high resolutions. This paper demonstrates three-dimensional imaging of a microfluidic device with nanometric depth precisions and high SNR. We demonstrate imaging of microelectrodes of nanometric thickness patterned on glass substrate and the microfluidic channel. Three-dimensional imaging of a transparent PDMS optomicrofluidic channel, fluid flow, and live yeast cell flow in this channel has been demonstrated using DHM. We also quantify the average velocity of fluid flow through the channel. In comparison to any conventional bright-field microscope, the 3D depth information in the images illustrated in this work carry much information about the biological system under observation. The results demonstrated in this paper prove the high potential of DHM in imaging optofluidic devices; detection of pathogens, cells, and bioanalytes on lab-on-chip devices; and in studying microfluidic dynamics in real time based on phase changes.
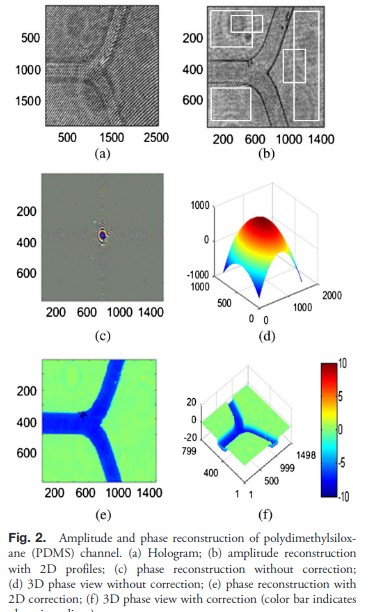
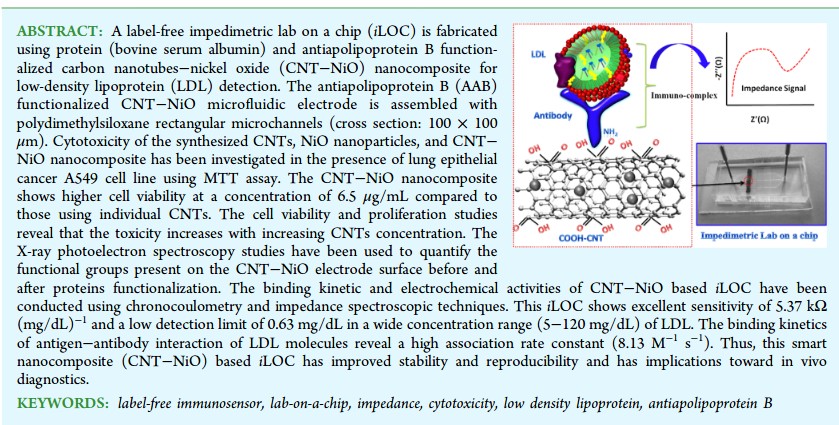
Abstract
Cardiovascular diseases (CVDs) are the leading causes of death worldwide. Cardiac troponin I (cTnI) is a cardiac biomarker exploited extensively for early diagnosis of acute myocardial infarction (AMI). We report a highly sensitive microfluidic biosensor for the electrochemical detection of human cTnI in real patient samples. In this device, a patterned mesoporous nickel vanadate hollow-nanosphere modified chitosan (Ch-Ni3V2O8) was integrated with a microfluidic structure. Owing to its excellent redox activity and biocompatibility, larger surface, and tunable oxidation states (V5+, V4+, and V3+), the Ch-Ni3V2O8 matrix was used to functionalize the cardiac antibodies of troponin I (cAb) and amplify the electrochemical readouts. This device offered a high sensitivity of 38.88 μA ng−1 mL−1 cm−2 at a wide range of cTnI concentrations (0.005–100 ng mL−1). A low limit-of-detection of 5 pg mL−1 and high stability, high reproducibility and good selectivity were achieved due to integration of microfluidic elements with a hollow-nanosphere Ch-Ni3V2O8. This microfluidic device can be implemented to detect B-type natriuretic peptide, myoglobin, cardiac troponin C, and cardiac troponin T by functionalizing the specific antibody recognition elements and could be used for real point-of-care applications in biomedicine.
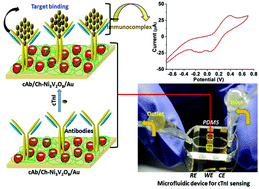
Abstract
We report the results of characterization of red blood cell (RBC) structure and its dynamics with nano- metric sensitivity using transport of intensity equation microscopy (TIEM). Conventional transport of intensity technique requires three intensity images and hence is not suitable for studying real-time dynamics of live bio- logical samples. However, assuming the sample to be homogeneous, phase retrieval using transport of intensity equation has been demonstrated with single defocused measurement with x-rays. We adopt this technique for quantitative phase light microscopy of homogenous cells like RBCs. The main merits of this technique are its simplicity, cost-effectiveness, and ease of implementation on a conventional microscope. The phase information can be easily merged with regular bright-field and fluorescence images to provide multidimensional (three- dimensional spatial and temporal) information without any extra complexity in the setup. The phase measure- ment from the TIEM has been characterized using polymeric microbeads and the noise stability of the system has been analyzed. We explore the structure and real-time dynamics of RBCs and the subdomain membrane fluctuations using this technique.
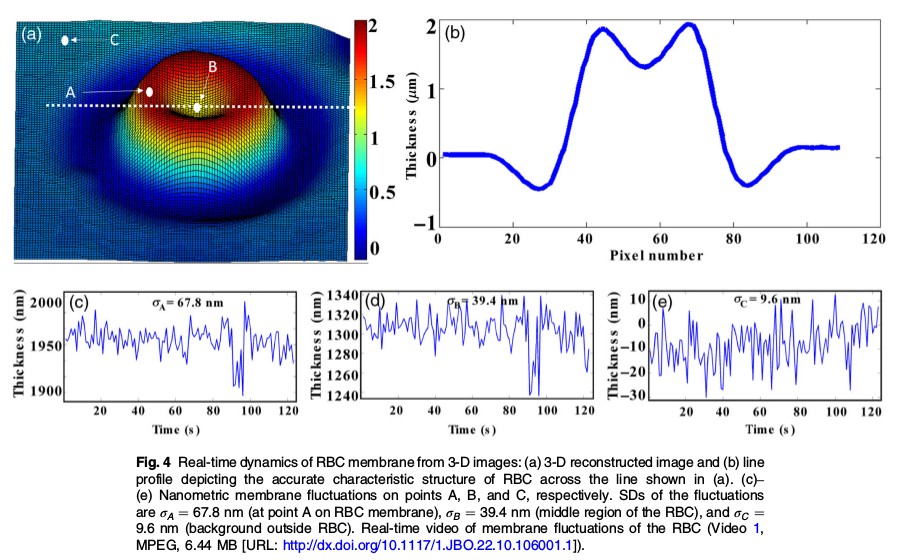
Abstract
Echocardiography is a widely used and cost-effective medical imaging procedure that is used to diagnose cardiac irregularities. To capture the various chambers of the heart, echocardiography videos are captured from different angles called views to generate standard images/videos. Automatic classification of these views allows for faster diagnosis and analysis. In this work, we propose a representation for echo videos which encapsulates the motion profile of various chambers and valves that helps effective view classification. This variety of motion profiles is captured in a large Gaussian mixture model called universal motion profile model (UMPM). In order to extract only the relevant motion profiles for each view, a factor analysis based decomposition is applied to the means of the UMPM. This results in a low-dimensional representation called motion profile vector (MPV) which captures the distinctive motion signature for a particular view. To evaluate MPVs, a dataset called ECHO 1.0 is introduced which contains around 637 video clips of the four major views: a) parasternal long-axis view (PLAX), b) parasternal short-axis (PSAX), c) apical four-chamber view (A4C), and d) apical two-chamber view (A2C). We demonstrate the efficacy of motion profile-vectors over other spatio-temporal representations. Further, motion profile-vectors can classify even poorly captured videos with high accuracy which shows the robustness of the proposed representation.
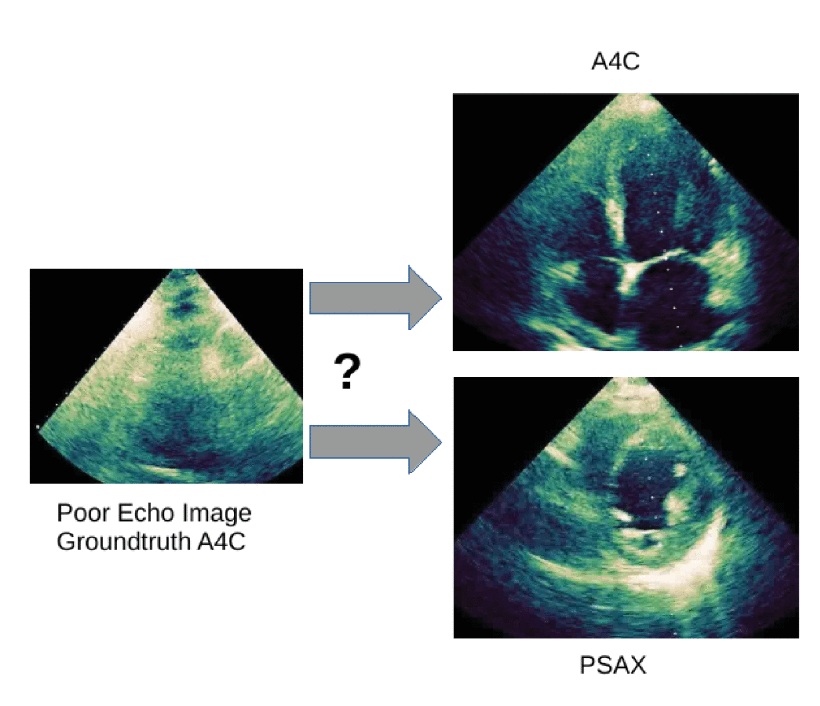
Details
Lymph node imaged on day 7 after injection. An en face OCT (a) and corresponding histology image (d) are shown. Lowscattering subcapsular regions have extended deeper into the node, and a more homogeneous highly scattering cortex is evident, as shown in (b), where follicular structures are no longer visible, as confirmed by histology in (e). An adjacent area reveals decreased optical scattering (yellow arrows), as seen in (c), from a histologically verified region of inflammation with aggregates of macrophages but no tumor cells (f). The green and yellow frames around the crosssectional OCT images (b and c, respectively) correspond to the horizontal lines of the same color in (a), indicating the positions of these cross sections relative to the en face OCT image. The highlighted and magnified histology areas (e and f) correspond to the left and right black-framed areas, respectively, on the complete en face lymph node section in (d).
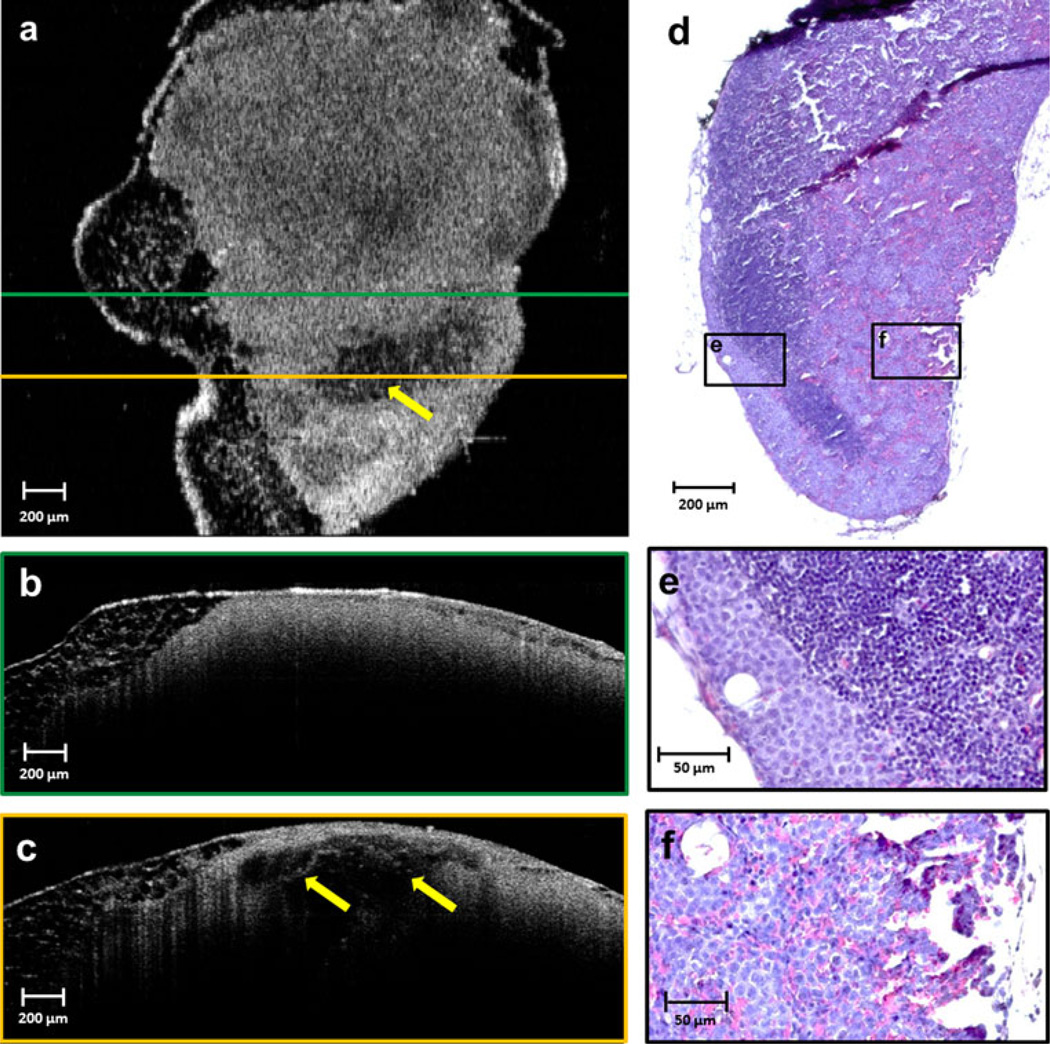
Abstract
In this study, protein-shell microspheres filled with a suspension of iron oxide nanoparticles in oil are demonstrated as multimodal contrast agents in magnetic resonance imaging (MRI), magnetomotive optical coherence tomography (MM-OCT), and ultrasound imaging. The development, characterization, and use of multifunctional multimodal microspheres are described for targeted contrast and therapeutic applications.
Figure: High-resolution real-time in vivo ultrasound images of rat mammary tumor and microbubble transients. a Tumor imaged with 15 MHz probe. b Doppler flow imaging identifying vascular region. c Transient increase in scattering (green trace) following commercial microbubble injection. d Rapid decrease in scattering (green trace) following delivery of rupture pulse (red region).
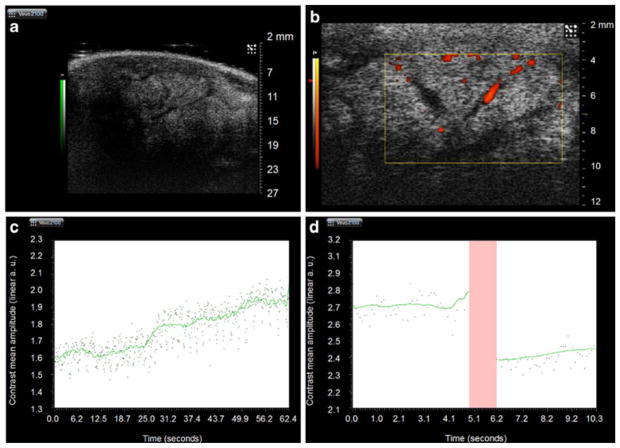
Abstract
We present a single disperser spectral imager that exploits recent theoretical work in the area of compressed sensing to achieve snapshot spectral imaging. An experimental prototype is used to capture the spatiospectral information of a scene that consists of two balls illuminated by different light sources. An iterative algorithm is used to reconstruct the data cube. The average spectral resolution is 3.6 nm per spectral channel. The accuracy of the instrument is demonstrated by comparison of the spectra acquired with the proposed system with the spectra acquired by a nonimaging reference spectrometer.
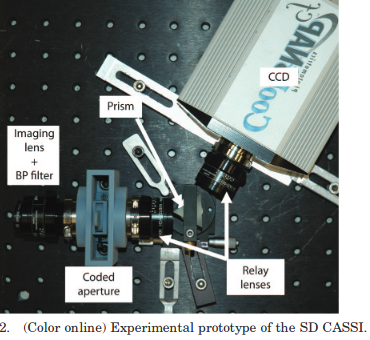
Abstract
This paper describes a single-shot spectral imaging approach based on the concept of compressive sensing. The primary features of the system design are two dispersive elements, arranged in opposition and surrounding a binary-valued aperture code. In contrast to thin-film approaches to spectral filtering, this structure results in easily-controllable, spatially-varying, spectral filter functions with narrow features. Measure- ment of the input scene through these filters is equivalent to projective measurement in the spectral domain, and hence can be treated with the compressive sensing frameworks recently developed by a number of groups. We present a reconstruction framework and demonstrate its application to experimental data.
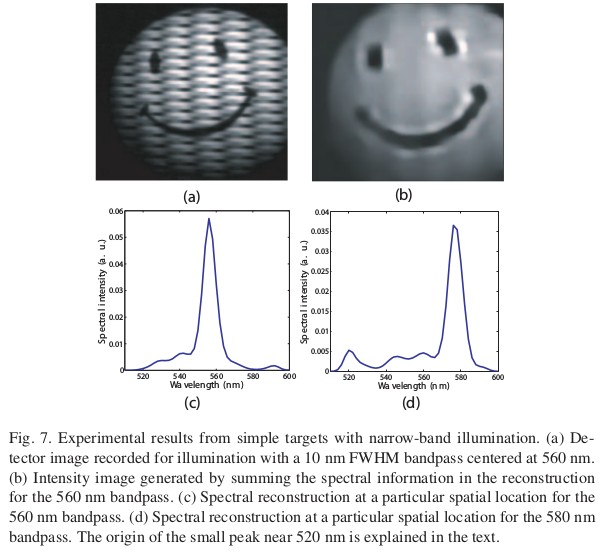
Abstract
We present an optical technique to image the frequency- dependent complex mechanical response of a viscoelastic sample. Three- dimensional hyperspectral data, comprising two-dimensional B-mode images and a third dimension corresponding to vibration frequency, were acquired from samples undergoing external mechanical excitation in the audio-frequency range. We describe the optical coherence tomography (OCT) signal when vibration is applied to a sample and detail the processing and acquisition techniques used to extract the local complex mechanical response from three-dimensional data that, due to a wide range of vibration frequencies, possess a wide range of sample velocities. We demonstrate frequency-dependent contrast of the displacement amplitude and phase of a silicone phantom containing inclusions of higher stiffness. Measurements of an ex vivo tumor margin demonstrate distinct spectra between adipose and tumor regions, and images of displacement amplitude and phase demonstrated spatially-resolved contrast. Contrast was also observed in displacement amplitude and phase images of a rat muscle sample. These results represent the first demonstration of mechanical spectroscopy based on B-mode OCT imaging. Spectroscopic optical coherence elastography (S- OCE) provides a high-resolution imaging capability for the detection of tissue pathologies that are characterized by a frequency-dependent viscoelastic response.
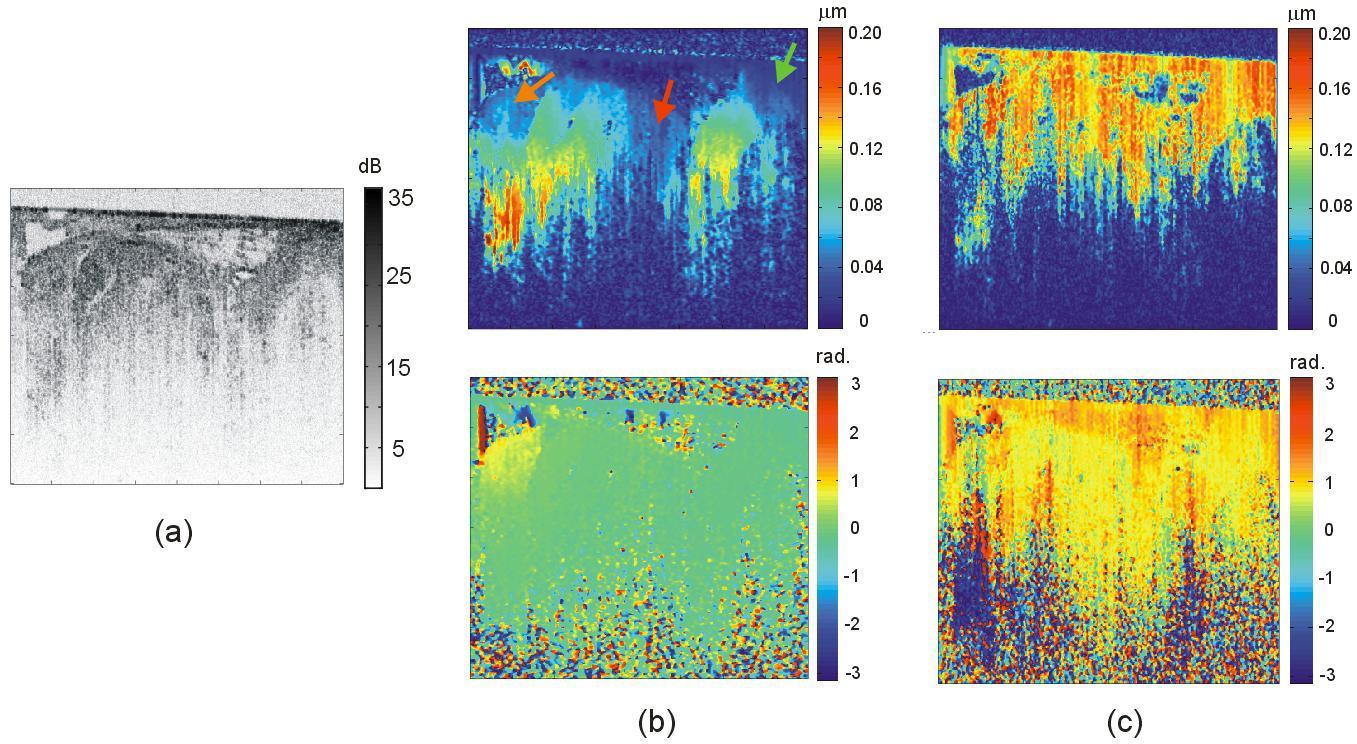
Figure:Complex mechanical response images for rat muscle tissue. (a) OCT image, and displacement amplitude (top) and phase images (bottom) at vibration frequencies of (b) 83 Hz and (c) 125 Hz. Image dimensions are 750 μm (optical depth) by 2 mm (lateral). Arrows in the displacement image at 83 Hz indicate regions with different OCE and OCT contrast.
Abstract
This paper describes a single-shot spectral imaging approach
based on the concept of compressive sensing. The primary features of
the system design are two dispersive elements, arranged in opposition
and surrounding a binary-valued aperture code. In contrast to thin-film
approaches to spectral filtering, this structure results in easily-controllable,
spatially-varying, spectral filter functions with narrow features. Measure-
ment of the input scene through these filters is equivalent to projective
measurement in the spectral domain, and hence can be treated with the
compressive sensing frameworks recently developed by a number of groups.
We present a reconstruction framework and demonstrate its application to
experimental data.
Figure MM-OCT principle. (A) Schematic of the experi-
mental setup. (B) TEM of the MNPs used in the study. (C)
Close-up of the sample arm with focusing lens, electro-
magnet, and specimen showing axial magnetomotive
displacements of MNPs and corresponding change in
the OCT interferogram. (D) (I) B-mode image through
a MNP-silicone phantom. (II–IV) Representative zoomed-
in images of M-mode scans along the dashed red line
marked in (I) showing magnetomotion in the sample
on application of a (II) sinusoidal, (III) pulse, and (IV)
sweep of frequencies of the magnetic field.
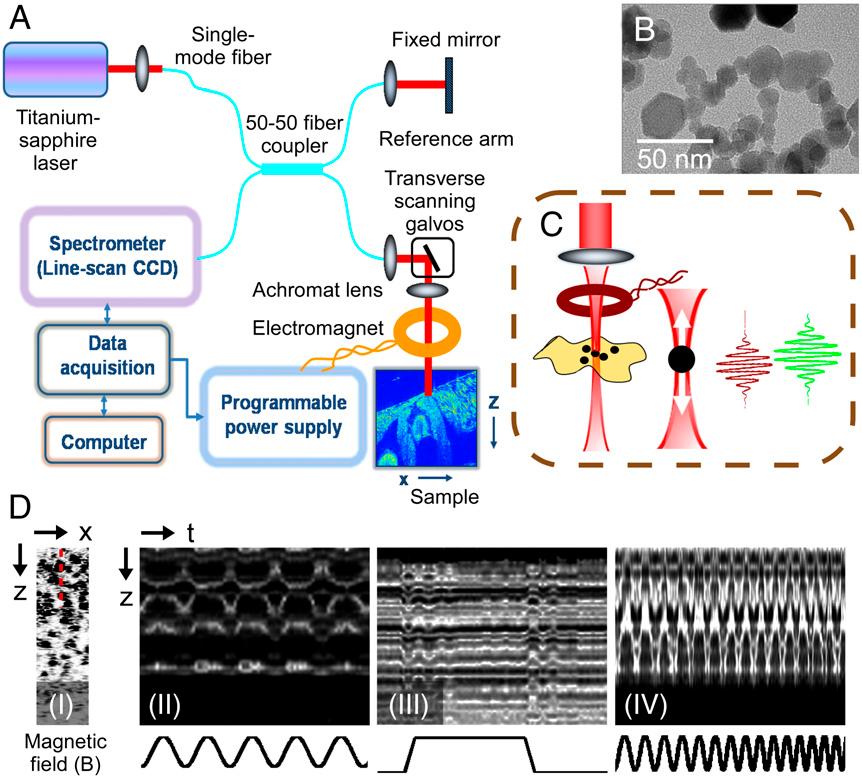
Abstract
A dynamic spectral-domain optical coherence elastography (OCE) imaging technique is reported. In this technique, audio-frequency compressive vibrations are generated by a piezoelectric stack as external excitation, and strain rates in the sample are calculated and mapped quantitatively using phase-sensitive spectral-domain optical coherence tomography. At different driving frequencies, this technique provides contrast between sample regions with different mechanical properties, and thus is used to mechanically characterize tissue. We present images of a three-layer silicone tissue phantom and rat tumor tissue ex vivo, based on quantitative strain rate. Both acquisition speed and processing speed are improved dramatically compared with previous OCE imaging techniques. With high resolution, high acquisition speed, and the ability to characterize the mechanical properties of tissue, this OCE technique has potential use in non-destructive volumetric imaging and clinical applications.
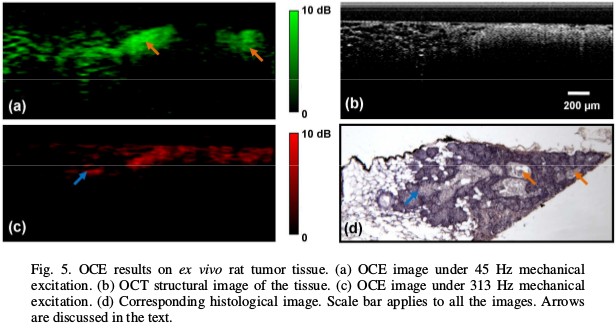
Books
- P Panta, CW Lu, P Kumar, TS Ho, H Sheng-Lung, P Kumar, R John, Optical Coherence Tomography: Emerging in Vivo Optical Biopsy Technique for Oral Cancer, Springer (2019)
- Renu John and Stephen A. Boppart, “Scattering, absorption, and modulating nanoprobes in Nano-Biophotonics, McGraw-Hill, New York, (2010).
Patent
- Apparatus and Methods for Label-Free Morphological Evaluation of Human Sperm
Press Coverage
- In vivo magnetomotive optical molecular imaging using targeted magnetic nanoprobes, PNAS 107 8085-8090 (2010)
- Magnetism puts nanoparticles on the move , Coverage by www. futurity.org
- New Imaging Method uses Magnetic Nanoparticles that Move, Beckman News letter April 2010.
- In vivo magnetomotive optical molecular imaging using targeted magnetic nanoprobes, OCT NEWS 2010